What is a qubit in quantum computing
Quantum computing is a fascinating field with the potential to change how we process information. The concept of a qubit is crucial to this cutting-edge technology. Unlike standard computer bits, qubits use quantum physics concepts to provide incredible computational capability. Qubits may exist in several states at the same time by using quantum superposition and entanglement, allowing for parallel processing and exponential gains in computer speed.
In this blog article, we’ll look at what qubits are and the interesting qualities that make them the foundation of quantum computing.
The Basics of Quantum Computing
A. Quantum Computing
Quantum computing is a new branch of study that uses quantum mechanics principles to alter the way we process and manipulate information. The concept of qubits, which are the building elements of quantum information, is central to quantum computing. Unlike traditional bits, which can only represent a 0 or a 1, qubits can exist in several states at the same time, according to a characteristic known as superposition. Because of this extraordinary property of qubits, quantum computers may do sophisticated calculations and solve problems that are now intractable for classical computers. Qubits enable considerably faster and more powerful computing capabilities by utilizing the phenomena of superposition, entanglement, and interference.
ad
B. Quantum Mechanics and Quantum Information
Quantum mechanics is a field of physics that describes the behavior of matter and energy at the atomic and subatomic levels. It provides a mathematical framework for comprehending and forecasting the probabilistic nature of particles, their wave-like properties, and their interactions. Quantum physics, which enables the manipulation and measurement of quantum states, is the cornerstone of quantum computing.
Quantum information is the encoding, processing, and transmission of information utilizing quantum physics principles. It makes use of quantum systems’ unique features, including as superposition and entanglement, to store and manipulate information in quantum bits, or qubits. Quantum information theory investigates the use of quantum systems for tasks such as secure communication, quantum cryptography, and quantum computation. Quantum information, by leveraging the strength of quantum mechanics, offers new possibilities for information processing that go beyond the capabilities of classical systems.
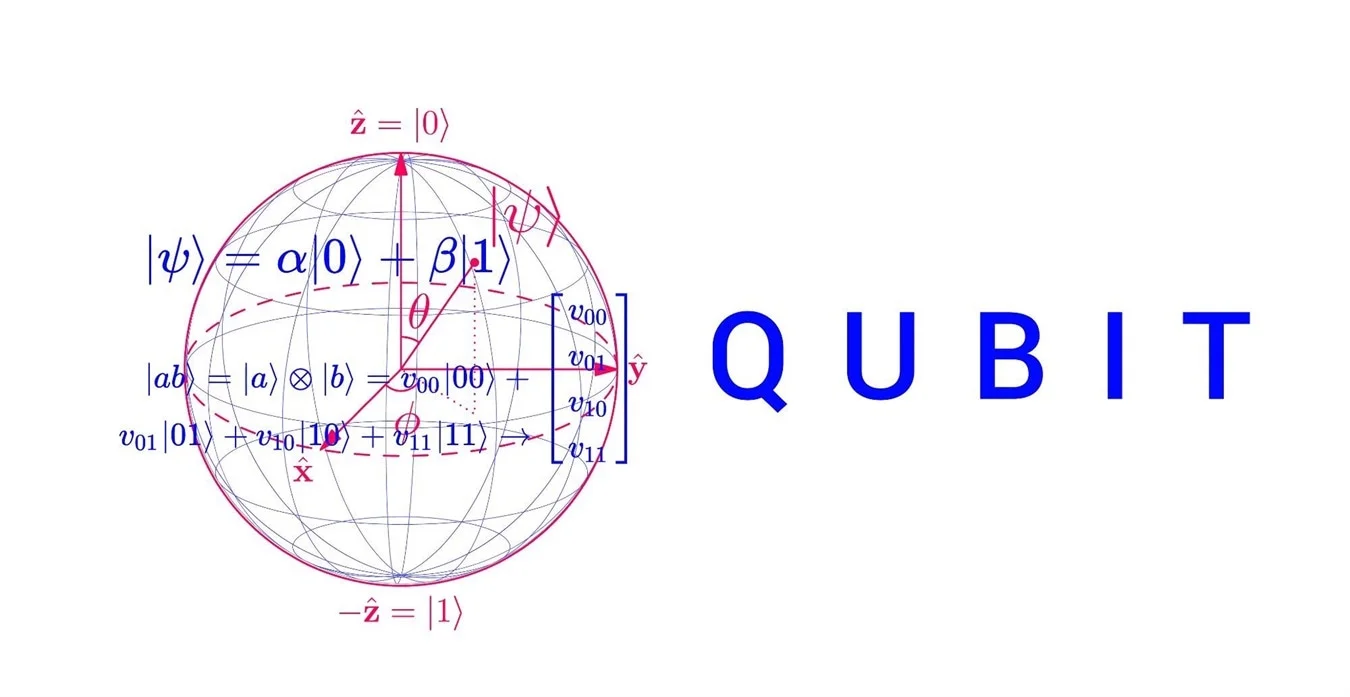
What is a qubit in quantum computing?
The fundamental unit of information in quantum computing is the qubit, short for quantum bit. While classical bits can only represent a 0 or a 1, qubits can exist in a state that is a superposition of both. This distinguishing feature derives from quantum superposition principles, which indicate that a qubit can be in a mixture of 0 and 1 until it is measured, at which point it collapses into one of the two states. The ability of qubits to exist in several states at the same time is what provides quantum computers with their enormous processing power. Furthermore, qubits can get entangled with other qubits, resulting in complex quantum states that allow for parallel computing and elaborate quantum algorithms. Understanding the nature of qubits is crucial for comprehending the potential of quantum computing and the groundbreaking advancements it promises to bring.
Types of qubits
In quantum computing, various physical systems can be utilized as qubits, the fundamental units of quantum information. Different types of qubits have been developed, each relying on distinct properties and technologies. Some common types of qubits include:
- Electron Spin Qubits: These qubits utilize the spin states of individual electrons to encode and process quantum information. Manipulating the spin of electrons trapped in quantum dots or defects in solid-state materials allows for quantum computations.
- Superconducting Qubits: Superconducting circuits, consisting of Josephson junctions and microwave resonators, are used as qubits. They rely on the behavior of superconducting materials at extremely low temperatures and exhibit quantum properties like superposition and coherence.
- Topological Qubits: Topological qubits rely on exotic properties of certain materials to create robust and stable qubits. They take advantage of the unique characteristics of anyons, specialized quasiparticles, to encode and manipulate quantum information.
- Photon Qubits: Photon-based qubits utilize the quantum properties of light particles to encode and process information. Photons can be manipulated using various techniques, such as linear optics or nonlinear interactions, to perform quantum computations.
- Ion Trap Qubits: In this approach, individual ions are trapped and manipulated using electromagnetic fields. The internal energy levels of ions serve as qubit states, and their interaction allows for the implementation of quantum gates and computations.
These are just a few examples of the diverse types of qubits employed in quantum computing. Each type has its own advantages and challenges, and ongoing research aims to enhance their performance and scalability for practical quantum computing applications.
Quantum Gates and Quantum Operations
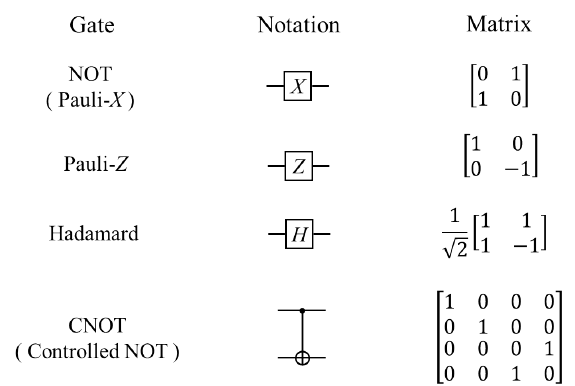
A. Single-Qubit Gates: Single-qubit gates are quantum operations that act on individual qubits, allowing for the manipulation and transformation of quantum states. Examples of single-qubit gates include Pauli gates (X, Y, Z) and the Hadamard gate, which enable operations such as flipping, rotating, and creating superposition in a single qubit.
B. Two-Qubit Gates: Two-qubit gates are quantum operations that operate on pairs of qubits, enabling interactions and entanglement between qubits. The CNOT (controlled-NOT) gate is a widely used two-qubit gate that flips the target qubit based on the state of the control qubit. The SWAP gate exchanges the states of two qubits. These gates form the foundation for building more complex quantum circuits and performing quantum computations involving multiple qubits.
C. Quantum Circuit Simulation: Quantum circuit simulation involves the use of quantum circuit simulators to predict and analyze the behavior of quantum circuits. These simulators allow researchers and developers to model and simulate quantum operations, explore the effects of different gates and measurements, and analyze the outcomes of quantum computations. Quantum circuit simulation is a valuable tool for understanding and optimizing quantum algorithms before implementing them on physical quantum hardware.
Quantum Algorithms and Applications
- Shor’s Algorithm: Shor’s algorithm is a significant quantum algorithm that has the potential to efficiently factor large numbers. This algorithm poses a significant threat to RSA cryptography, which relies on the difficulty of factoring large numbers for secure communication and data protection. Shor’s algorithm demonstrates the potential of quantum computers to solve problems that are intractable for classical computers.
- Grover’s Algorithm: Grover’s algorithm is a quantum algorithm that offers a quadratic speedup for searching an unsorted database compared to classical algorithms. It provides an efficient solution to the unstructured search problem and has implications for optimization and database search applications. Grover’s algorithm showcases the power of quantum computing in solving problems with exponential speedup over classical approaches.
- Quantum Machine Learning: Quantum machine learning is an emerging field that explores the synergy between quantum computing and machine learning. It encompasses quantum-inspired algorithms, such as quantum support vector machines and quantum neural networks, as well as techniques for quantum data processing and quantum data analysis. Quantum machine learning has the potential to revolutionize fields such as pattern recognition, optimization, and data analysis by leveraging the unique properties of quantum systems.
Quantum error correction
Introduction to Quantum Error Correction: Quantum error correction is essential in protecting quantum information from errors and decoherence. Due to the delicate nature of quantum systems, errors can occur during quantum operations, leading to the loss or corruption of quantum information. Quantum error correction techniques aim to detect and correct these errors, preserving the integrity of the encoded quantum states and enabling reliable quantum computations.
Quantum Error Correction Codes: Quantum error correction codes are mathematical schemes designed to encode quantum information in a way that allows for the detection and correction of errors. Stabilizer codes and surface codes are two prominent examples of quantum error correction codes. Stabilizer codes use a set of stabilizer operators to detect and correct errors, while surface codes utilize a two-dimensional lattice structure to encode and protect quantum information.
Fault-Tolerant Quantum Computing: Fault-tolerant quantum computing is a field of research focused on designing quantum computing systems that can perform reliable quantum computations despite the presence of noise and errors. It involves the development of error-correcting codes, fault-tolerant quantum gates, and fault-tolerant quantum circuits. The goal of fault-tolerant quantum computing is to overcome the limitations imposed by quantum decoherence and errors, paving the way for practical and scalable quantum computers.
Quantum Hardware Platforms
- IBM Quantum: IBM Quantum is a leading quantum hardware platform that offers access to quantum processors, software tools, and a cloud-based environment for quantum computing. IBM provides a range of quantum processors with increasing qubit counts, allowing users to run quantum experiments and explore quantum algorithms. The platform also offers software development kits (SDKs) like Qiskit, which enable users to program and simulate quantum circuits.
- Google Quantum: Google has made significant advancements in quantum hardware, primarily focusing on superconducting qubits. Their quantum hardware initiatives include the Quantum Supremacy experiment, where they demonstrated the ability of a quantum computer to solve a specific problem faster than classical computers. Google continues to push the boundaries of quantum hardware research and development.
- Microsoft Quantum: Microsoft takes a unique approach to quantum computing by focusing on topological qubits. They aim to leverage the topological properties of certain materials to create robust and error-resistant qubits. Microsoft is actively involved in research, development, and collaboration to advance the field of quantum computing. They also provide development tools like the Microsoft Quantum Development Kit, which includes a quantum programming language and simulation capabilities.
- Rigetti Computing: Rigetti Computing specializes in superconducting qubit-based quantum processors. They offer access to their quantum hardware through their Quantum Cloud Services, allowing users to run quantum programs on their quantum processors. Rigetti’s Forest software development kit provides tools and resources for programming and simulating quantum circuits, enabling researchers and developers to explore and experiment with quantum algorithms
Pros and Cons
Pros | Cons |
---|---|
|
|
The Power of Qubits in Quantum Computing
The fundamental unit of information in quantum computing is the qubit, which is a quantum analogue to classical bits. While traditional bits can only indicate a 0 or a 1, qubits provide much more. They can be realized using various quantum systems, such as electrons or photons, and have features that are distinct from classical bits.
A classical bit can be physically represented by a recognizable and binary state, similar to a light bulb being either on or off. A qubit, on the other hand, can exist not only in the classical states of 0 and 1, but also in a superposition, embodying both states at the same time. This ability to be in numerous states at the same time is a result of quantum superposition, a fundamental property of quantum mechanics.
Qubits can also be subjected to incompatible measurements and get entangled with other qubits, resulting in complex quantum states. This entanglement phenomenon allows qubits to have a strong connection, allowing the manipulation of one qubit to instantly alter the state of another, regardless of their physical distance.
Qubits, by utilizing the properties of superposition, interference, and entanglement, expand the computational capabilities and information processing beyond the restrictions of classical bits. The discipline of quantum computing seeks to exploit these unique qualities in order to create innovative algorithms and solve complicated problems that are unsolvable for traditional computers. With ongoing advancements in qubit technologies, scientists are exploring a wide range of physical systems to serve as qubits, each with its own advantages and challenges in realizing the full potential of quantum computing.
ad
Comments are closed.